Epidemiologic considerations
Before appropriate therapy can be given for an infectious disease, consideration of epidemiologic factors is essential. This section does not fully discuss the epidemiology (the determinants, occurrence, distribution, and control of health and disease) of infectious diseases. However, a number of basic principles and historical points are worth emphasizing.
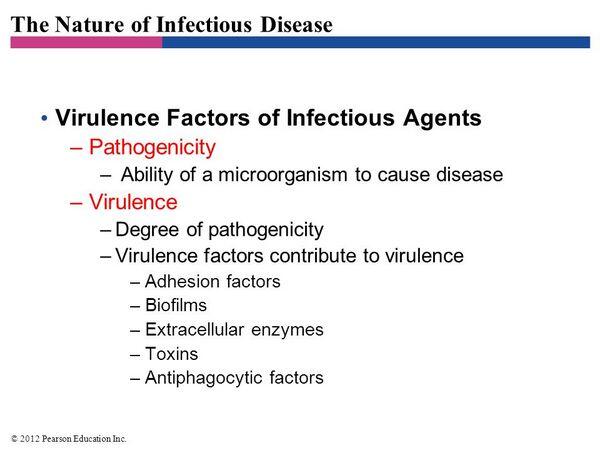
Basic principles of transmission.
An infectious disease results from the interaction between an infectious agent and a susceptible host. The agent may originate from a source external to the host (exogenous infection), or, because of changes in the agent–host relationship, a normally occurring, usually innocuous, microbial agent on skin or mucosal surfaces can produce disease (endogenous infection). The relationship among the agent, its transmission, and the host represents the chain of infection. In general, the greatest risk for developing infections in the immunocompetent individual is related to acquisition of pathogenic microorganisms (e.g., Shigella. Among immunocompromised patients (e.g., leukemic patients undergoing chemotherapy), endogenous flora (e.g., staphylococci or enteric gram-negative bacilli such as Escherichia coli) are more likely the cause of disease.
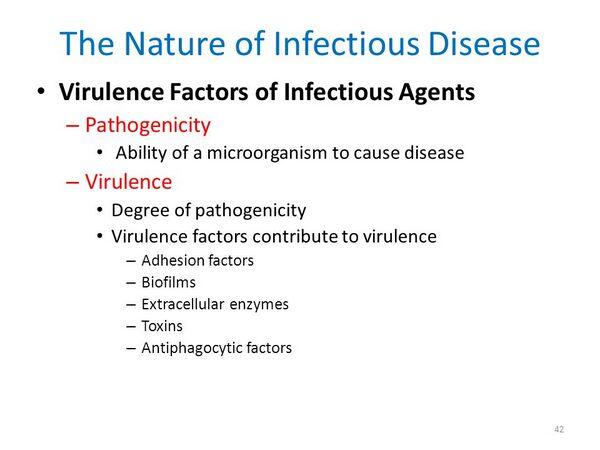
Acquisition
Humans are first exposed to microorganisms at birth during passage through the vagina. Thereafter, acquisition, carriage, and clearance of microorganisms are basic facts of life. There are four main routes of acquisition of microorganisms: 1) contact, 2) inhalation, 3) common-vehicle, and 4) vector-borne. Table Transmission of Specific Diseases outlines the source, route of acquisition, and portal of entry of microorganisms causing certain specific diseases. The route for contact acquisition involves direct transfer of the agent to the susceptible host from person to person or via fomite or droplet. For transmission by inhalation, the agent is airborne in small particles (<5 mm diameter). In contrast, contact droplet spread occurs when large droplets (≥5 mm diameter) are physically transmitted directly onto surfaces of the respiratory tract. Streptococcal and measles infections are examples of droplet-spread disease. They usually occur when multiple persons are exposed to a single index patient who comes in close contact with susceptible hosts. In contrast, transmission by inhalation occurs when susceptible hosts are exposed to aerosols containing microbes. Direct-proximity contact is not necessary. Tuberculosis is an example of spread by inhalation of contaminated aerosols from human to human; psittacosis and Q fever represent transmission from an infected animal to human by inhalation transmission. In the last-mentioned [Author, Q fever only? changed from in this instance], aerosolized Coxiella burnetii organisms or spores can be windborne miles from the source, and inhalation by a susceptible host results in Q fever.
Table Transmission of Specific Diseases | |||||||||||||||||||||||||||||||||
|
A common inanimate vehicle usually serves to transmit an agent to multiple hosts. The most frequently involved common vehicles are food and water, which can transmit a number of different pathogens (e.g., enteric bacillary pathogens and hepatitis A). Contaminated needles are an important common vehicle for transmitting human immunodeficiency virus, hepatitis B, and hepatitis C virus amon0g injection drug users.
For some agents, maturation and multiplication through intermediate hosts (e.g., arthropod vectors) are necessary preliminaries for transmission to humans. Vector-borne diseases (e.g., malaria) are termed biologically transmitted diseases.
Patient information
A complete history is often the key to making an etiological diagnosis of an infectious disease. Where the patient has been, what he has done, and with whom he has done it is a succinct summary. Important information can be gained from a consideration of age, gender, place of residence, family, and other personal contacts (including the history of these contacts with disease), occupation, hobbies, contacts with animals, travel history, exposure to parenteral drugs or blood products, sexual habits, dietary habits, other active medical problems, and medications. Obviously, the thoroughness with which this information is collected is tailored to the particular situation. The medical equivalent to a surgical “second look” is to retake the history. Since the physician has not made a diagnosis at this point, careful attention to items that seem insignificant is important.
In hospital-acquired infections, important points of epidemiologic information that should be sought include the types of infectious complications that are associated with different procedures or conditions in general; the particular microorganisms that may be specific to the particular institution in which you are practicing (e.g., Serratia marcescens resistant to gentamicin); previous or current antimicrobial therapy; the concomitant use of other medications that might alter host defense or the manifestations of disease (e.g., corticosteroids or other immunosuppressive agents); history of transfusion or parenteral use of medication; the incidence and type of infections in other patients or personnel who have been in contact with the patient. The decisions involved in empirical selection of antimicrobials to treat hospital-acquired infections are usually more complex than those for community-acquired infections. These decisions must be founded on an accurate data base that is continually reviewed for new trends in infections in a particular hospital. In general, this task is assigned to an infection surveillance and control committee in the hospital.
Infectivity
The sequence of acquisition followed by colonization and multiplication is termed the infectivity of the organism. Individuals harbor a varied population of microorganisms that have different potentials to produce disease. The endogenous flora periodically include a number of organisms that are commonly described as virulent and called pathogens. The names are apt because the organisms have been clearly identified as major causative agents of disease.
For example, strains of Neisseria meningitidis are a leading cause of bacterial meningitis in infants, children, and adults but are often found in the nasopharynx of healthy individuals (carriers) of all ages (e.g., 25 to 40% of normal young adults). Carriers can be properly thought of as being “colonized” with a potentially virulent organism which, because of host and other immunologic factors, is contained to a mucosal surface.
In fact, this carrier state is probably crucial for eliciting protective immunity. Other “pathogens” that can be carried for long periods without their hosts developing disease include pneumococci, Streptococcus pyogenes, Staphylococcus aureus, salmonellae, and many viruses including herpes group agents. When host defenses are altered, infections may develop not only with these recognized pathogens but with other organisms that are usually considered harmless.
In the absence of selected host defense factors, some pathogens are so virulent that, when acquired, they virtually always produce disease. For example, in a classic epidemiologic study of persons who lacked protective antibody and were colonized with rubeola virus in the upper respiratory tract, the attack rate of measles exceeded 90%. [Author, Original rubeola, is rubella better?]
Knowledge of the infectivity and virulence of microorganisms is important in determining the likelihood of infection. Mere documentation of the presence of a potential pathogen is not sufficient reason to institute treatment unless disease is present or the threat of disease is great. Nowhere is this more important than in the intubated ventilated patient. Potentially pathogenic microorganisms are almost always isolated from cultures of respiratory secretions in this setting.
Principles
The decision to institute antibiotic therapy depends upon consideration of all clinical and laboratory data designed to answer the question: Is this an infection or is this colonization? Knowledge of the infectivity and virulence of a microorganism is crucial.
Virulence factors
Microbial factors that influence the severity of the infection include the size of the infective dose, the duration of exposure of the host to the microbe, competition from surrounding flora, and the virulence of infecting microorganism (this includes a variety of factors including the ability to cause infection at low doses, to resist host defenses, and to elaborate compounds that cause tissue injury). For example, the pathogenesis of ascending, nonobstructive Escherichia coli pyelonephritis in women with anatomically normal urinary tracts exemplifies a number of important characteristics. These include both organism and host factors. The organism factors are presence of pili, certain K antigen types, and secretion of hemolysin and colicin V. Host factors include a short urethra in women (rendering them more susceptible than men to urinary tract infection during childhood and early adulthood). In addition, the presence of the Lewis blood group nonsecretor and recessive phenotype is found more commonly among women with recurrent urinary tract infections, suggesting a genetic predisposition. This infection may be viewed as the culmination of a sequence of events mediated by specific determinants of microbial virulence.
Key points
Microbial factors that influence severity of infection include the following:
- Inoculum size
- Duration of exposure of host to microbe
- Competition from surrounding flora
- Virulence of the pathogen.
In summary, the pathogenic process of microbial disease is orderly and requires sequential steps for disease. Virulence factors enable the microbial pathogen to establish, proliferate, damage, and disseminate in specific niches of the host. These determinants of bacterial virulence are under the control of a regulatory system that enables the microorganism to adapt to and overcome local host environments. Relatively little is known about either the specific environmental signals (e.g., temperature, pH, calcium, iron, amino acids) to which these systems respond or the rationale for these responses. The regulatory system usually involves a pair of proteins: One protein acts as a sensor of environmental stimuli and transmits a signal to the other protein, usually by means of phosphorylation; the second protein, once stimulated, acts to regulate gene expression.
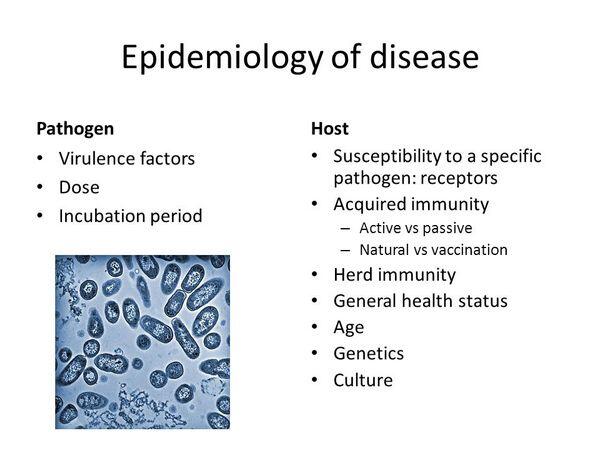
There may be many regulatory systems in the genetic elements of the microorganism that respond independently to a variety of environmental stimuli. However, coordinated control of a group of operons is under the domain of a regulon. A regulon provides a means by which many genes can respond in a coordinated fashion to a particular environmental stimulus. The regulon exerts its regulatory functions by trans-acting regulatory loci that exert positive or negative expression on a number of virulence factors, e.g., vir in Bordetella pertussis and virR in Shigella. These determinants mediate their regulatory function at the level of transcription. Another mechanism by which bacteria can regulate expression of virulence and thereby evade host defenses is DNA rearrangements. A well-studied example of this is the expression of flagellin genes in Salmonella typhimurium. By this mechanism, S. typhimurium expresses either H1 or H2 flagellins, thereby evading possible host antibody responses. Other examples include Trypanosoma brucei in which subpopulations of the parasite have antigenically different forms of a major variant-specific glycoprotein at the cell surface. Individual trypanosomes are capable of producing more than 100 different VGGs during the course of an infection.
Table Uropathogenesis of Escherichia coli Pyelonephritis | |||||||||||||||
|
This theme of modification of surface structure is a common method of avoiding the host immune response. Two recent observations help explain various aspects of bacterial virulence. The first of these is the concept of pathogenicity islands. These are discrete segments of DNA that encode virulence traits and appear to have a foreign origin. These pieces of foreign DNA are often missing in closely related nonvirulent bacteria. The second observation is that of type III or contact-dependent secretion: So far type III secretion has been described only in gram-negative bacteria. This mechanism allows bacteria to secrete toxins or other products while in direct contact with a host cell.
Microbial pathogens may evade the host’s immune system by adapting to intracellular survival where they are hidden from phagocytic cells as well as from complement and antibody. While microorganisms are within the cell, they may reside in host cell phagosomes or endosomes or, through lysis of the endosome, escape into the cytoplasm. Salmonella typhi, for example, may reside transiently in intestinal epithelial cells before systemic spread. Chlamydia trachomatis, Mycobacterium tuberculosis, and Legionella pneumophila reside in endosomal vacuoles, whereas species of Rickettsia and Listeria monocytogenes escape the phagosome and reside in the cytoplasm. In the case of L. monocytogenes, the nonmotile intracellular bacteria can move within the host cell’s cytoplasm by polymerizing actin filaments in a manner that propels the bacteria and permits intracellular spread. In all these cases, the microbe is able to subvert or redirect host cell biological processes; in fact, microbes have become probes for elucidating fundamental cell biological processes associated with organelle trafficking and intracellular signaling.
Those microorganisms that replicate within phagosomes must either prevent acidification and subsequent fusion of microorganism-laden phagosomes with secondary lysosomes (phagosome–lysosome fusion) or exhibit a survival strategy for coping with low pH conditions and increased levels of reduced oxygen radicals and nitric oxide. Generally, the process of phagocytosis initiates a series of biochemical events that include acidification of the microorganism laden endosome and the activation of Rab proteins associated with organelle trafficking (Rab 5 and 7) that facilitate the fusion of these endosomes with secondary lysosomes. Leishmania and Mycobacteria can survive acidified phagosomes, whereas Chlamydia and Legionella cannot. Recent studies indicate that pathogens use different strategies in subverting phagosomes to hospitable domiciles. In the case of L. pneumophila, an intracellular parasite of protozoa in aquatic environments and an opportunistic human pulmonary pathogen, the bacteria block phagosome–lysosome fusion by redirecting the bacteria-laden phagosome to the endoplasmic reticulum. Here, the endoplasmic reticulin envelops the phagosome, forming what is termed a replicative phagosome that is now permissive for bacterial multiplication. It is evident that the Legionellae modify the phagosome so that it becomes refractory to secondary lysosomes, while recruiting nonlysosomal vesicles, mitochondria, and ribosomes.
Although intracellular parasites are protected from the effects of antibody and complement, these organisms are still susceptible to the action of the cytotoxic T lymphocytes and natural killer cells (natural killer cells). In many cases, a small number of highly conserved proteins, stress proteins or heat shock proteins (Hsps), are processed and presented by infected host cells in major histocompatibility class I or II surface molecules, and these antigens are recognized by T lymphocytes. These stress protein-derived peptides, together with secretion of interleukins, promote a T helper 1 (Th1) type (cytoxic) immune response. Several recent studies have shown that members of the Hsp60 and Hsp70 families of stress-induced proteins can induce production of IL-1 and IL-12. Moreover, Hsp60 has been shown to bind to the surface of macrophages and to signal through protein kinase C IL-1 production. Whereas IL-1 plays a general role in promoting a fever response and activating the immune system, IL-12 plays a major role in the immune response of most intracellular parasites as well as infections caused by noninvasive microbial pathogens. IL-12 activates T cells to produce γ-interferon (IFN-γ) and tumor necrosis factor β. It is noteworthy that IFN-γ production leads to further stimulation of IL-12 production by phagocytic cells. In addition, IFN-γ also downregulates transferrin receptors leading to decreased intracellular levels of iron, as well as activating the production of nitrous oxide. Taken together, these interactions generally provide an effective response to invasive microbial parasites by either killing them outright or inhibiting their intracellular multiplication. Immunocompromised individuals are at greater risk for developing severe disease because of failure of the cellular immune system to produce those necessary cytokines. Although some consideration has been given to administering IL-12 and other cytokines to immunocompromised individuals, it should be emphasized that most of the cytokines are not systemic molecules, but their action is required locally.
In some instances, changes in host receptors determine the virulence of a microorganism. Streptococcus pneumoniae colonizes 20 to 30% of humans, but only a few develop invasive disease. In the human lung both the opaque and the disease-causing transparent pneumococci adhere to alveolar cells. However, when a viral respiratory tract infection is present, the host cytokines activate platelet-activating factor on the surface of lung epithelial cells. The transparent pneumococci can adhere to this receptor and can invade via the pulmonary capillaries or lymphatic vessels.
Principles
Understanding the molecular pathogenesis of bacterial virulence offers the possibility of new therapeutic strategies that could not have been considered without such information.
Host factors
The ability of a microorganism to produce disease in the host is a function of the interplay between the virulence of the microorganism and host factors. Host factors that interfere with a microorganism’s ability to colonize, proliferate, invade, or injure include nonspecific and specific defense mechanisms (Table Host Factors Associated with Defense Against Microorganisms). Nonspecific responses represent primitive protective mechanisms that are not specifically directed at a particular microorganism and that do not involve immunologic memory. In contrast, specific host defense mechanisms involve lymphocytes that kill microorganisms specifically or that elicit antibody production. Specific antibodies bind to selected microbial antigens and, in coordination with the classic complement system, eventually kill microorganisms. These specific host defense mechanisms involve immunologic memory and are important for host immunity on subsequent exposure or infection by microorganisms. Defects in any of the host’s defensive weapons increase the host’s susceptibility to infection. It must be stressed that although a majority of infections are associated with microbial evasion of host factors, the elicited host responses (e.g., cytokines) to microorganisms can account for considerable host injury.
Table Host Factors Associated with Defense Against Microorganisms | ||||||||||||||||
|
Principles
In considering the pathogenesis of infection, it is crucial to assess the defects in host defenses that contributed to disease. One goal of treatment is to correct these abnormalities so that a successful clinical outcome will occur and disease will not worsen or recur after therapeutic intervention. Conversely, when host defense mechanisms contribute to injury during infection, modulation of host factors may be required for successful outcomes.
Some important host factors that are not generally considered in discussions of immunity include normal flora tissue, tropism of the microorganism, and cytokines. Microorganisms that usually produce disease must come in contact with or penetrate the skin or mucosal surfaces. The integrity of skin and mucous membrane barriers constitutes a major line of defense against microorganisms.
The majority of infections among leukemic patients undergoing chemotherapy occur only when these surfaces are broken, thereby allowing endogenous flora to invade deeper structures and cause disease. Under normal conditions, the flora that usually colonize epithelial surfaces (e.g., skin and gastrointestinal tract) also protect the host from microbial invasion by exogenous pathogens. The nonspecific mechanisms of this protection include competition for the same nutrients, competition for host receptors for colonization, and production of bacteriocins that are toxic to other microbes. The result of these mechanisms is to limit the ability of a “foreign microbe” to colonize and proliferate on these surfaces (also known as colonization resistance). Normal flora are influenced by a number of factors including diet, hormones, sanitary conditions, hygienic habits, and exposure to toxins and antibiotics. Indiscriminate use of antimicrobials is a common problem; the resulting changes in endogenous flora may produce deleterious effects in the host. Antibiotic concentrations at these epithelial surfaces can be sufficient to kill microorganisms directly or to affect their physiology. The overall effects of antibiotics on normal flora are to decrease their number or their ability to persist on these surfaces. These changes enable potential pathogens to colonize the epithelial surfaces.
Normal flora also are crucial for continuous “priming” of the immune system. The continuous influx of products from normal flora across epithelial surfaces represents a constant priming signal for macrophages to process these substances. In germ-free animals and newborns, there is an overall lack of antigen processing by the host, as exemplified by the low levels of class II histocompatibility (DR) molecule expression on macrophages. However, once endogenous flora are established on epithelial mucosal surfaces, there are marked increases in expression of DR molecules in macrophages. Therefore, the constant exposure of host macrophages and T cells to antigens from indigenous flora enables the host to be primed for immune responses. The maintenance of the normal flora is crucial for health.
Principles
Antibiotics can have deleterious effects on the host by eliminating or adversely affecting the beneficial effects of the normal flora.
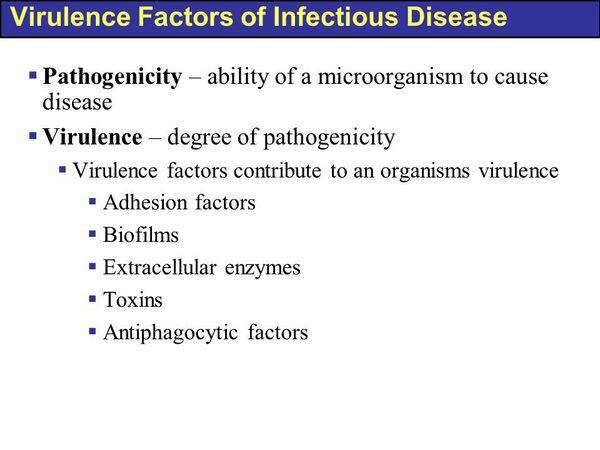
The ability of microorganisms to colonize specific epithelial surfaces is linked to the presence of microbial adhesins and host receptors that permit attachment. Most microorganisms, whether endogenous or pathogenic, preferentially colonize certain tissues; this phenomenon is referred to as tissue tropism. Host receptors for attachment of microorganisms vary depending on the tissue (e.g., esophagus versus colon), cellular mixture (e.g., epithelial cells versus stromal constituents), and conditions of health (e.g., during menstruation) or disease (e.g., following viral and bacterial infections). Table Selected E. coli Adhesins and Host Receptors lists adhesins used by Escherichia coli and their corresponding host receptors.
These receptor compounds usually are located on epithelial surfaces and enable the microorganism to colonize these surfaces. For example, type 1 pili of uropathogenic E. coli strains can bind to uroepithelia because d-mannose is bound to these cells. However, the host possesses a natural defense factor to prevent epithelial mucosal binding by this adhesion under normal conditions. Renal tubular cells secrete the Tamm-Horsfall uromucoid, a highly mannosylated glycoprotein. This uromucoid interferes with uroepithelial attachment of bacteria with this mannose adherence specificity, because it binds bacterial adhesins when the microorganism is in the urinary stream. The binding of the bacterial adhesin by d-mannose moieties in the uromucoid represents an important normal host factor that prevents bacteriuria. There are a number of other host excretory and secretory substances (e.g., saliva, tears, fibronectin, mucus) that hinder colonization of mucosal surfaces by microbes. A number of normal processes (e.g., gastrointestinal peristalsis, the ciliary movement of the respiratory epithelia, cough, and micturition) then remove these unattached microorganisms from the host.
The use of soluble receptor analogs to prevent microorganism attachment to target host cells is a new therapeutic strategy. For example, administration of soluble CD4 molecule prevents human immunodeficiency virus attachment to T-helper cells in vitro, thus abrogating viral invasion of these cells. The usefulness of this strategy in clinical conditions requires complete inhibition of virus attachment to host cells and effective clearance of the microorganism from the host. This exciting new direction in disease prevention has been made possible only by elucidating the basis of the attachment of microorganisms to the host at a molecular level (namely, tropism).
Table Selected E. coli Adhesins and Host Receptors | ||||||||||||||||
|
Principles
The ability of microorganisms to attach to cells is crucial to the pathogenesis of microbial disease. Interference at this step has therapeutic potential.
Considerable progress within the last decade has elucidated the chemical basis of regulation of host immunity. It appears that cytokines are responsible for many of the host’s responses to microorganisms, accounting for acute inflammation and long-term immunity.
These proteins are produced locally by a variety of stromal cells. They are secreted in small quantities and have short half-lives (of seconds to minutes). They act within a complicated network producing a cascade of events. Several inflammatory cytokines (e.g., IL-1, IL-6, and tumor necrosis factor-α) appear to contribute to physiologic events that can result in death. Numerous studies have demonstrated the correlation between elevated concentrations of inflammatory cytokines and death due to bacterial septicemia. Further work is required to elucidate the actions of specific cytokines and to develop approaches to modulating their effects. At a different level, various types of immune deficiency states are associated with different infectious syndromes.